Mitochondria in the heart - The heart, a tireless powerhouse that sustains life, relies on the microscopic workhorses known as mitochondria. As our understanding of these essential organelles advances, so does the potential for groundbreaking therapeutic strategies in cardiovascular disease. In this blog post, we will explore the diverse roles of mitochondria in the cardiovascular system, the genetic factors influencing mitochondrial dysfunction in heart diseases, and the latest developments in therapies and research tools targeting these cellular dynamos.
Embark on a journey through the heart's cellular landscape and witness the fascinating world of mitochondrial dynamics, communication, and function. Delve into the intricacies of ATP production, calcium regulation, and cell death pathways, as well as the molecular mechanisms underlying mitochondrial dysfunction in conditions like dilated cardiomyopathy, specifically related to mitochondria in the heart. Discover cutting-edge therapeutic approaches and research models that are propelling the field of mitochondrial biology forward, bringing hope for a healthier future.
Short Summary
- Mitochondria are essential for supplying energy to the heart, regulating calcium in cardiomyocytes and controlling cell death pathways.
- Genetic mutations can have a significant impact on mitochondrial function and lead to various cardiovascular diseases.
- Research is ongoing into therapies targeting mitochondria as well as tools such as cardiac organoids, which may revolutionize our understanding of mitochondrial dysfunction
Mitochondria in the Heart: It's Functions in the Cardiovascular System
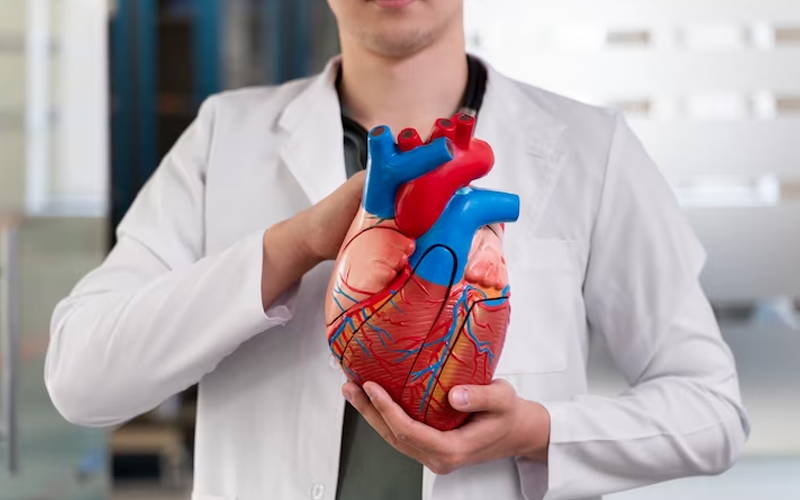
ATP Production and Energy Metabolism
Calcium Regulation in Cardiomyocytes
Mitochondria and Cell Death Pathways
Mitochondrial Dynamics and Communication
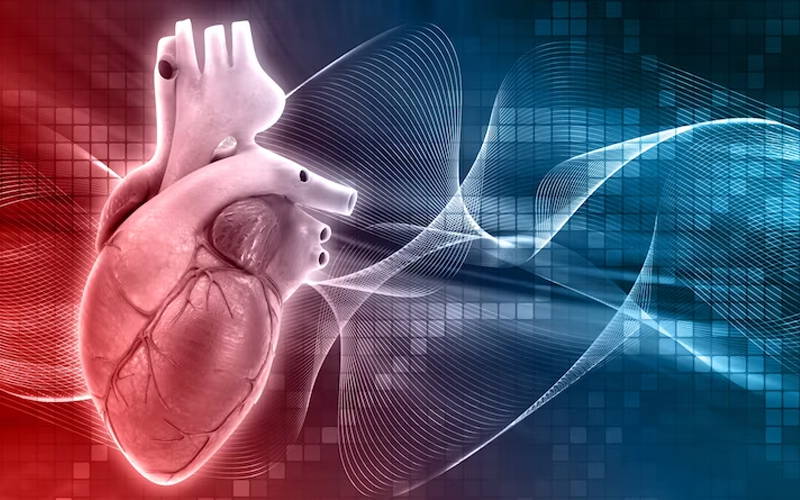
Fusion, Fission, and Mitophagy
Intercellular Communication and Signaling
Genetic Factors and Mitochondrial Dysfunction in Heart Diseases
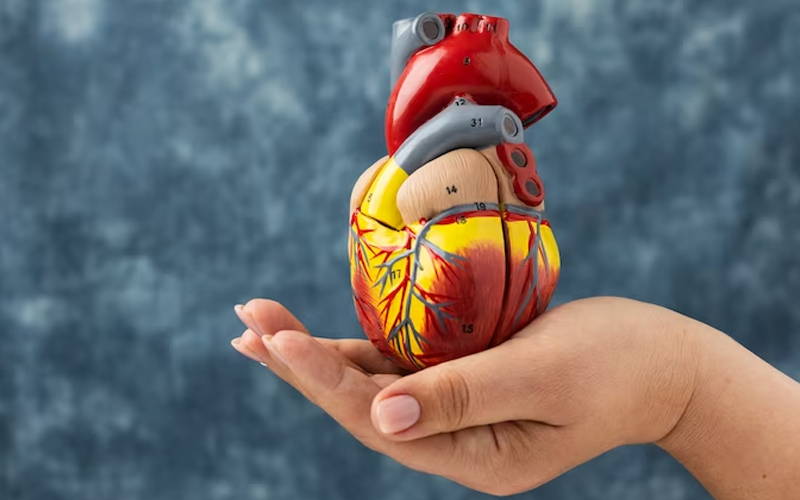
Genetic Mutations Affecting Mitochondrial Function
Mitochondrial Dysfunction in Dilated Cardiomyopathy
Therapeutic Approaches Targeting Mitochondria
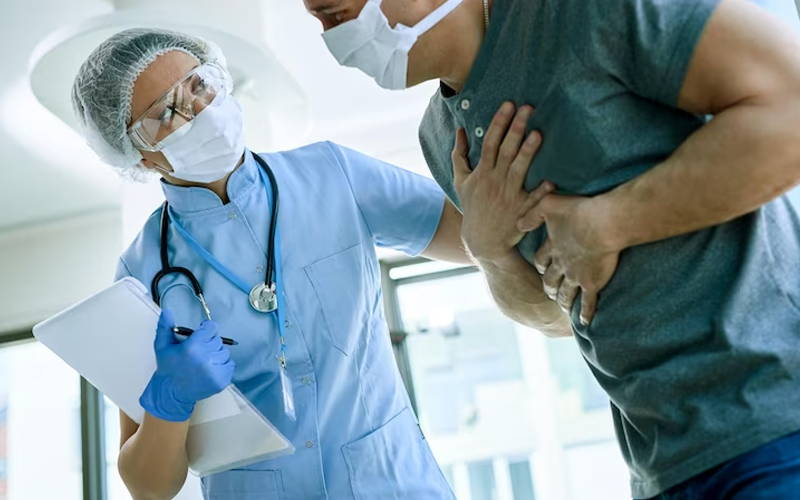
Strategies for Enhancing Mitochondrial Function
Advances in Mitochondria-Targeted Therapies
Tools and Techniques for Studying Mitochondria in Cardiac Diseases
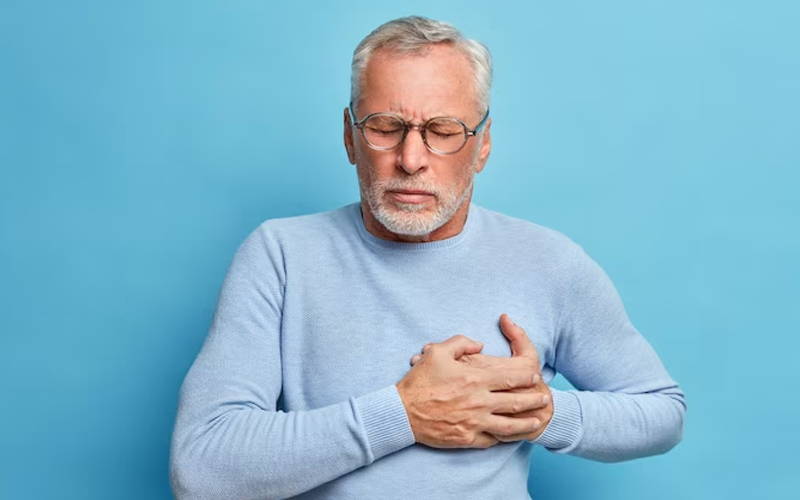
Genomic, Proteomic, and Metabolomic Approaches
Cardiac-Specific Organoids as Research Models
Summary
Frequently Asked Questions
What is the role of the mitochondria in the heart?
Does the heart contain mitochondria?
What are the symptoms of mitochondria heart disease?
Why does the heart have the most mitochondria?
What are the 3 main functions of the mitochondria?
Recent Posts
Author
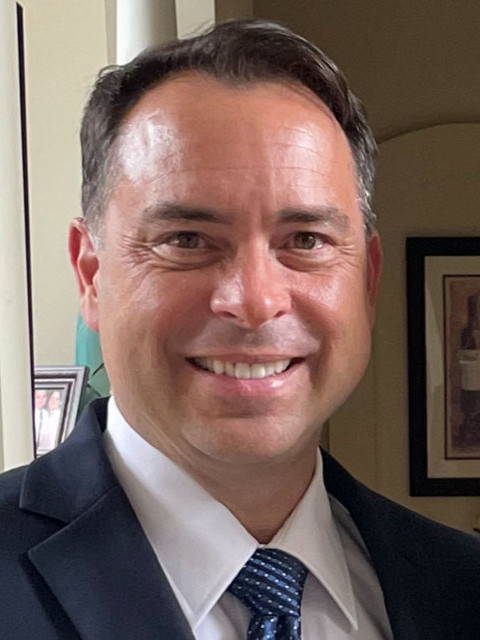
Michael has a diverse set of skills and passions, with a full-time career as an airline pilot and a dedicated focus on health and fitness consulting. He understands the importance of balancing a busy lifestyle with maintaining a healthy mind and body, and is committed to helping others achieve the same success. Michael's expertise in health and fitness is not just limited to physical training, but also extends to nutrition, stress management, and overall wellbeing. He takes a holistic approach to health and fitness, helping clients to achieve their goals in a sustainable and fulfilling way. With a strong desire to inspire and motivate others, Michael is always ready to share his time and knowledge with those who seek his guidance. Whether in the air or on the ground, Michael is dedicated to helping others live their best lives.